Doing More and Doing Better with Fiber-Reinforced Shotcrete
Design and testing comparison
by Antoine Gagnon and Marc Jolin
Over the years, fiber-reinforced shotcrete (FRS) has proven to be a very effective and versatile material.
It plays an essential and often unique role in ground support systems in mines and many tunneling projects, and it makes possible the safe and economical construction of various civil structures. Simply put, FRS performs well in situations where installing conventional reinforcing bars or cast fiber-reinforced concrete (FRC) would be complex and tedious or simply unsafe. However, we believe that FRS does not get the consideration it deserves. This article will discuss how FRS can achieve more recognition and how we can do better in exploiting its impressive properties and capturing its full potential.
by Antoine Gagnon and Marc Jolin
Over the years, fiber-reinforced shotcrete (FRS) has proven to be a very effective and versatile material.
It plays an essential and often unique role in ground support systems in mines and many tunneling projects, and it makes possible the safe and economical construction of various civil structures. Simply put, FRS performs well in situations where installing conventional reinforcing bars or cast fiber-reinforced concrete (FRC) would be complex and tedious or simply unsafe. However, we believe that FRS does not get the consideration it deserves. This article will discuss how FRS can achieve more recognition and how we can do better in exploiting its impressive properties and capturing its full potential.
What Makes FRS So Interesting
FRS is a composite material created by pneumatically projecting a concrete mixture and fibers onto a surface. More formally, a mixture of cement, water, aggregate, and admixtures are combined with discrete, discontinuous filaments comprised of a material with a high tensile strength and/or a high toughness. The performance of FRS obviously comes from the quality of the shotcrete (its constituents and process) and the performance of the fibers, but also from the interaction between the fibers and the shotcrete matrix.
Unfortunately, the latter aspect is too often overlooked when designing an FRS mixture.
FRS is subject to the actions that are specific to shotcrete. In the process of placing shotcrete, the material is sprayed at high velocity and builds up on a receiving surface. The consolidation energy of the material impacting the surface produces compaction that allows shotcrete ingredients to work together efficiently through a strong packing density.1 Also, the impact tends to give a preferential orientation to the fibers that is generally beneficial in the structure because the fibers are mainly oriented in a plane that is perpendicular to the nozzle axis and parallel to the surface sprayed.2,3 We recently studied this in our Shotcrete Laboratory at Université Laval, Québec City, QC, Canada, using the fiber orientation factor α, which is the average, for all possible fiber orientations, of the projected fiber length in the tensile stress direction to the fiber length itself.4 For a standard wet-mix shotcrete mixture, we found that α = 0.648 for tension in a plane perpendicular to the nozzle and α = 0.223 for tension in a plane perpendicular to the nozzle axis. Because cracks generally form perpendicularly to the former plane, it is clear that most of the fibers in FRS are in the best position to effectively transfer stress across a crack.
The flexibility of the shotcrete placement process also allows the buildup of a uniform thickness of FRS on surfaces that are naturally uneven. Because it is designed to adhere to vertical and even overhead surfaces, shotcrete does not need to be supported by formwork. For example, it is possible to follow the shape of an excavation without having to unnecessarily overfill the cavities. This is particularly cost- effective and allows for fast construction and fast reentry of work personnel in underground environments. Compared to other construction methods and other ground support systems, it is generally considered much faster and much simpler.5
Getting the most out of FRS is about finding the right “composite” for the situation or application considered. It is also a question of finding the optimal interaction between fibers and shotcrete. This varying combination allows for a wide range of possibilities in terms of mixtures and applications. Fortunately, our level of understanding has improved over the past decades—the rheology and the placement process of shotcrete are now better controlled, which opens the doors to many new applications. It is also possible to use the information that applies more generally to FRC by adapting it to the context of the shotcrete placement process. After all, FRS is FRC.
FRS is a composite material created by pneumatically projecting a concrete mixture and fibers onto a surface. More formally, a mixture of cement, water, aggregate, and admixtures are combined with discrete, discontinuous filaments comprised of a material with a high tensile strength and/or a high toughness. The performance of FRS obviously comes from the quality of the shotcrete (its constituents and process) and the performance of the fibers, but also from the interaction between the fibers and the shotcrete matrix.
Unfortunately, the latter aspect is too often overlooked when designing an FRS mixture.
FRS is subject to the actions that are specific to shotcrete. In the process of placing shotcrete, the material is sprayed at high velocity and builds up on a receiving surface. The consolidation energy of the material impacting the surface produces compaction that allows shotcrete ingredients to work together efficiently through a strong packing density.1 Also, the impact tends to give a preferential orientation to the fibers that is generally beneficial in the structure because the fibers are mainly oriented in a plane that is perpendicular to the nozzle axis and parallel to the surface sprayed.2,3 We recently studied this in our Shotcrete Laboratory at Université Laval, Québec City, QC, Canada, using the fiber orientation factor α, which is the average, for all possible fiber orientations, of the projected fiber length in the tensile stress direction to the fiber length itself.4 For a standard wet-mix shotcrete mixture, we found that α = 0.648 for tension in a plane perpendicular to the nozzle and α = 0.223 for tension in a plane perpendicular to the nozzle axis. Because cracks generally form perpendicularly to the former plane, it is clear that most of the fibers in FRS are in the best position to effectively transfer stress across a crack.
The flexibility of the shotcrete placement process also allows the buildup of a uniform thickness of FRS on surfaces that are naturally uneven. Because it is designed to adhere to vertical and even overhead surfaces, shotcrete does not need to be supported by formwork. For example, it is possible to follow the shape of an excavation without having to unnecessarily overfill the cavities. This is particularly cost- effective and allows for fast construction and fast reentry of work personnel in underground environments. Compared to other construction methods and other ground support systems, it is generally considered much faster and much simpler.5
Getting the most out of FRS is about finding the right “composite” for the situation or application considered. It is also a question of finding the optimal interaction between fibers and shotcrete. This varying combination allows for a wide range of possibilities in terms of mixtures and applications. Fortunately, our level of understanding has improved over the past decades—the rheology and the placement process of shotcrete are now better controlled, which opens the doors to many new applications. It is also possible to use the information that applies more generally to FRC by adapting it to the context of the shotcrete placement process. After all, FRS is FRC.
The Consideration That It Deserves
Because FRS helps us achieve so much in so many contexts, it seems appropriate to take some time to make sure it is specified to perform at its best. As for all concrete, this means that special care should be given to the choice of ingredients, mixture proportions, testing methods, and design approaches.
For shotcrete, the right choice of ingredients is essential to achieve the desirable properties in both fresh and hardened states. The aggregate size distribution is a key parameter in this matter; a good distribution will make a mixture that is both pumpable and sprayable. This is particularly important for FRS, as the introduction of fibers tends to reduce the workability of shotcrete. Whenever possible, it is better to focus on good base materials to reach the right pumping and spraying behaviors rather than having to rely only on chemical admixtures and risk incorrect use and unnecessary costs.6,7
FRS should be considered a “dynamic” material because the proportions of its components may change during the placement process. The effect of rebound—shotcrete material that bounces away from the surface—is responsible for this shift of proportions. In fact, this phenomenon is usually minimized by adequate tuning of the equipment and by maintaining a proper consistency of the material. This is always true for shotcrete, but it can also affect the fiber content when working with FRS because fibers behave like elongated aggregates and can bounce off the surface.8 Indeed, one must understand that the final fiber content of the in-place material is usually different from the initial fiber content.2,9
FRS is used in a wide range of contexts with different loading conditions. It is sometimes used in challenging environments where the loading conditions are complex; deep mines and highly stressed ground openings are great examples. Therefore, it is essential to give appropriate consideration to the test method that will be used for the evaluation of FRS. This is particularly true considering the number of standard test methods available:
ASTM C1399/C1399M, “Standard Test Method for Obtaining Average Residual-Strength of Fiber-Reinforced Concrete”;
ASTM C1550, “Standard Test Method for Flexural Toughness of Fiber Reinforced Concrete (Using Centrally Loaded Round Panel)”;
ASTM C1609/1609M, “Standard Test Method for Flexural Performance of Fiber-Reinforced Concrete (Using Beam with Third-Point Loading)”;
UNE 83-515, “Fibre Reinforced Concrete. Determination of Cracking Strength, Ductility and Residual Tensile
Strength. Barcelona Test”10;
EN 14488-511 for determination of energy absorption capacity of fiber-reinforced slab specimens;
EN 1465112 for measuring the flexural tensile strength; “EFNARC Three Point Bending Test on Square Panel with Notch”13;
Norwegian round panel test14; and
Grimstad and Barton.15
It is crucial to understand what information will be produced and how it will be used. Will it be used to compare with a design value, or will it be used as a quality indicator? Because different test methods do not test samples under the same conditions, it is generally hard to compare test methods directly, even though it may seem convenient. Although a given test method may show an increase in performance of a mixture, a second test method may not. This means that performance results from one test method are often not comparable to results from another test method. Finding the right test method to be used and the right way to use the information are essential steps for ensuring correct conclusions and appropriate decisions.
Particularly when working with FRS, it is important to consider and understand the idea behind the testing procedure selected. Testing a material is the same as asking a question. For example: How does this material react under the specific conditions of this test method? Subsequently, an answer to the question can be retrieved from the results, and this answer can be shared or used for design or performance evaluation.
Essentially, it is crucial to understand the test (question) that is being run (asked) and the result (answer) that is collected.
For example, the compressive strength is a commonly evaluated characteristic of concrete, and it is generally a good indicator of the quality of the placement of shotcrete and the quality of its ingredients. In some applications, the compressive strength can be the only performance specification for shotcrete.16 However, it is generally not a good indicator of the performance of FRS. In the typical range of fiber contents found, the compressive strength of FRS is not affected by the fibers.1 Also, having the strongest concrete mixture (that is, the highest compressive strength) is not the correct approach to obtaining an FRS with the best properties (highest energy absorption, for example). In fact, the behavior of this composite comes from the interaction between the fiber and the concrete. Indeed, in an FRS composite system, a fiber that slowly pulls out of the concrete will dissipate more energy than a fiber that breaks because it is too strongly anchored. Focusing on making a strong(er) concrete is not necessarily the best way to reach an ideal composite action.
Finally, as for concrete in general, the attention given to the sampling procedure is not only necessary but also beneficial in making the appropriate decisions. As it was explained previously, the placement process of shotcrete has a strong effect on the characteristics of the in-place material. Thus, creating test specimens that are representative of the actual structure is an important aspect of the design steps, quality
control, and quality assurance. This is particularly important for FRS because the rebound of fibers and their orientation in the structure can affect the performance of the material.
Luckily, many documents focus on this aspect and give guidance on the sampling procedure of shotcrete.
As a matter of fact, ACI technical committees have published multiple documents that can guide engineers, researchers, concrete producers, and contractors in the way they approach FRS. First, ACI 506R, “Guide to Shotcrete,”16 is a general reference document that provides tools on the appropriate production, placement, and testing of shotcrete. Second, ACI 506.1R, “Guide to Fiber-Reinforced Shotcrete,”8 gives specific details about the use of fibers in shotcrete.
Finally, ACI Committee 544, Fiber Reinforced Concrete, offers a number of documents on the subject of FRC, including a lot of information that applies to FRS.17-23
Because FRS helps us achieve so much in so many contexts, it seems appropriate to take some time to make sure it is specified to perform at its best. As for all concrete, this means that special care should be given to the choice of ingredients, mixture proportions, testing methods, and design approaches.
For shotcrete, the right choice of ingredients is essential to achieve the desirable properties in both fresh and hardened states. The aggregate size distribution is a key parameter in this matter; a good distribution will make a mixture that is both pumpable and sprayable. This is particularly important for FRS, as the introduction of fibers tends to reduce the workability of shotcrete. Whenever possible, it is better to focus on good base materials to reach the right pumping and spraying behaviors rather than having to rely only on chemical admixtures and risk incorrect use and unnecessary costs.6,7
FRS should be considered a “dynamic” material because the proportions of its components may change during the placement process. The effect of rebound—shotcrete material that bounces away from the surface—is responsible for this shift of proportions. In fact, this phenomenon is usually minimized by adequate tuning of the equipment and by maintaining a proper consistency of the material. This is always true for shotcrete, but it can also affect the fiber content when working with FRS because fibers behave like elongated aggregates and can bounce off the surface.8 Indeed, one must understand that the final fiber content of the in-place material is usually different from the initial fiber content.2,9
FRS is used in a wide range of contexts with different loading conditions. It is sometimes used in challenging environments where the loading conditions are complex; deep mines and highly stressed ground openings are great examples. Therefore, it is essential to give appropriate consideration to the test method that will be used for the evaluation of FRS. This is particularly true considering the number of standard test methods available:
ASTM C1399/C1399M, “Standard Test Method for Obtaining Average Residual-Strength of Fiber-Reinforced Concrete”;
ASTM C1550, “Standard Test Method for Flexural Toughness of Fiber Reinforced Concrete (Using Centrally Loaded Round Panel)”;
ASTM C1609/1609M, “Standard Test Method for Flexural Performance of Fiber-Reinforced Concrete (Using Beam with Third-Point Loading)”;
UNE 83-515, “Fibre Reinforced Concrete. Determination of Cracking Strength, Ductility and Residual Tensile
Strength. Barcelona Test”10;
EN 14488-511 for determination of energy absorption capacity of fiber-reinforced slab specimens;
EN 1465112 for measuring the flexural tensile strength; “EFNARC Three Point Bending Test on Square Panel with Notch”13;
Norwegian round panel test14; and
Grimstad and Barton.15
It is crucial to understand what information will be produced and how it will be used. Will it be used to compare with a design value, or will it be used as a quality indicator? Because different test methods do not test samples under the same conditions, it is generally hard to compare test methods directly, even though it may seem convenient. Although a given test method may show an increase in performance of a mixture, a second test method may not. This means that performance results from one test method are often not comparable to results from another test method. Finding the right test method to be used and the right way to use the information are essential steps for ensuring correct conclusions and appropriate decisions.
Particularly when working with FRS, it is important to consider and understand the idea behind the testing procedure selected. Testing a material is the same as asking a question. For example: How does this material react under the specific conditions of this test method? Subsequently, an answer to the question can be retrieved from the results, and this answer can be shared or used for design or performance evaluation.
Essentially, it is crucial to understand the test (question) that is being run (asked) and the result (answer) that is collected.
For example, the compressive strength is a commonly evaluated characteristic of concrete, and it is generally a good indicator of the quality of the placement of shotcrete and the quality of its ingredients. In some applications, the compressive strength can be the only performance specification for shotcrete.16 However, it is generally not a good indicator of the performance of FRS. In the typical range of fiber contents found, the compressive strength of FRS is not affected by the fibers.1 Also, having the strongest concrete mixture (that is, the highest compressive strength) is not the correct approach to obtaining an FRS with the best properties (highest energy absorption, for example). In fact, the behavior of this composite comes from the interaction between the fiber and the concrete. Indeed, in an FRS composite system, a fiber that slowly pulls out of the concrete will dissipate more energy than a fiber that breaks because it is too strongly anchored. Focusing on making a strong(er) concrete is not necessarily the best way to reach an ideal composite action.
Finally, as for concrete in general, the attention given to the sampling procedure is not only necessary but also beneficial in making the appropriate decisions. As it was explained previously, the placement process of shotcrete has a strong effect on the characteristics of the in-place material. Thus, creating test specimens that are representative of the actual structure is an important aspect of the design steps, quality
control, and quality assurance. This is particularly important for FRS because the rebound of fibers and their orientation in the structure can affect the performance of the material.
Luckily, many documents focus on this aspect and give guidance on the sampling procedure of shotcrete.
As a matter of fact, ACI technical committees have published multiple documents that can guide engineers, researchers, concrete producers, and contractors in the way they approach FRS. First, ACI 506R, “Guide to Shotcrete,”16 is a general reference document that provides tools on the appropriate production, placement, and testing of shotcrete. Second, ACI 506.1R, “Guide to Fiber-Reinforced Shotcrete,”8 gives specific details about the use of fibers in shotcrete.
Finally, ACI Committee 544, Fiber Reinforced Concrete, offers a number of documents on the subject of FRC, including a lot of information that applies to FRS.17-23
Laboratory Experience
Results from a recent research program well represent the affirmation by which different wet-mix shotcrete designs respond differently to different test procedures. In this series of experiments, three different FRS mixtures were tested following two test methods on panels that are commonly used in the industry for the design and testing of FRS: ASTM C1550 and EN 14488-5.11 In both test methods, a shotcrete panel is subjected at its center to a load controlled by deflection rate (Fig. 1). The peak load and energy absorption (toughness) of the FRS samples are measured in both of these procedures.
The concrete batches for all test panels had the same mixture proportions and steel fiber dosage of 25 kg/m3 (shown in Table 1). However, Mixtures A, B, and C incorporated Bekaert Dramix® 3D-45/35 BL, Dramix® 4D-65/35 BG, and Dramix® 5D-65/60 BG steel fibers (shown in Table 2). A priori, these fibers should create different behaviors with the same concrete mixture proportions, as the fibers’ geometries, tensile strengths, and anchoring systems are different. Based on the fibers’ properties, we would expect Mixture C to have better performance than Mixture B, and we would expect Mixture B to have better performance than Mixture A.
Results from a recent research program well represent the affirmation by which different wet-mix shotcrete designs respond differently to different test procedures. In this series of experiments, three different FRS mixtures were tested following two test methods on panels that are commonly used in the industry for the design and testing of FRS: ASTM C1550 and EN 14488-5.11 In both test methods, a shotcrete panel is subjected at its center to a load controlled by deflection rate (Fig. 1). The peak load and energy absorption (toughness) of the FRS samples are measured in both of these procedures.
The concrete batches for all test panels had the same mixture proportions and steel fiber dosage of 25 kg/m3 (shown in Table 1). However, Mixtures A, B, and C incorporated Bekaert Dramix® 3D-45/35 BL, Dramix® 4D-65/35 BG, and Dramix® 5D-65/60 BG steel fibers (shown in Table 2). A priori, these fibers should create different behaviors with the same concrete mixture proportions, as the fibers’ geometries, tensile strengths, and anchoring systems are different. Based on the fibers’ properties, we would expect Mixture C to have better performance than Mixture B, and we would expect Mixture B to have better performance than Mixture A.


Fig. 1: Setup for bending tests: (a) ASTM C1550; and (b) EN 14488-511
The results summarized in Table 3 show that the ASTM C1550 and EN 14488-511 test methods do not reflect identical increases in performance. Regardless of the absolute values of energy absorption, the trend is different from one test method to another. By normalizing the value of energy absorption at maximum deflection with Mixture A as a reference, it is possible to highlight this trend (Fig. 2). ASTM C1550 shows a lower increase in performance relative to the lowest value (Mixture A) compared to the increase shown with EN
14488-5.11 This shows that one FRS mixture could be preferred over another, depending on the test method used to characterize the material. The results also indicate that the design process could be affected, again depending on the test method used.
The results support the idea that, because of the loading conditions, some test methods tend to be more sensitive to the fiber type, the fiber dosage, the compressive strength of the concrete, or the interaction between the shotcrete and the fibers. In this case, the EN 14488-5 test method showed a clear strength advantage of Mixture C over the other mixtures, with a 110% increase with regard to Mixture A. In contrast, the ASTM C1550 test method shows only a 71% strength advantage of Mixture C over Mixture A. It is possible that, for that specific shotcrete mixture, the square panel on continuous support (EN 14488-511) is more sensitive to the fiber- anchoring system. These test method conditions could increase the deflection hardening behavior of Mixture B and Mixture C (both contain fibers with efficient anchoring systems). The anchor systems could also help to maintain a steady load capacity up to a 25 mm (1 in.) deflection, making the energy absorption value higher.
These conclusions mean that the choice of test method is important, as it can influence the decisions in different steps of a construction process, particularly during design. The test
The results summarized in Table 3 show that the ASTM C1550 and EN 14488-511 test methods do not reflect identical increases in performance. Regardless of the absolute values of energy absorption, the trend is different from one test method to another. By normalizing the value of energy absorption at maximum deflection with Mixture A as a reference, it is possible to highlight this trend (Fig. 2). ASTM C1550 shows a lower increase in performance relative to the lowest value (Mixture A) compared to the increase shown with EN
14488-5.11 This shows that one FRS mixture could be preferred over another, depending on the test method used to characterize the material. The results also indicate that the design process could be affected, again depending on the test method used.
The results support the idea that, because of the loading conditions, some test methods tend to be more sensitive to the fiber type, the fiber dosage, the compressive strength of the concrete, or the interaction between the shotcrete and the fibers. In this case, the EN 14488-5 test method showed a clear strength advantage of Mixture C over the other mixtures, with a 110% increase with regard to Mixture A. In contrast, the ASTM C1550 test method shows only a 71% strength advantage of Mixture C over Mixture A. It is possible that, for that specific shotcrete mixture, the square panel on continuous support (EN 14488-511) is more sensitive to the fiber- anchoring system. These test method conditions could increase the deflection hardening behavior of Mixture B and Mixture C (both contain fibers with efficient anchoring systems). The anchor systems could also help to maintain a steady load capacity up to a 25 mm (1 in.) deflection, making the energy absorption value higher.
These conclusions mean that the choice of test method is important, as it can influence the decisions in different steps of a construction process, particularly during design. The test
Table 1:
Mixture proportions for Mixtures A, B, and C
Mixture proportions for Mixtures A, B, and C
Material | Quantity |
Cement, kg/m3 (lb/yd3) | 377 (635) |
Silica fume, kg/m3 (lb/yd3) | 29 (49) |
Fly ash, kg/m3 (lb/yd3) | 72 (121) |
Fine aggregate, kg/m3 (lb/yd3) | 1060 (1787) |
Coarse aggregate, kg/m3 (lb/yd3) | 568 (957) |
Water, kg/m3 (lb/yd3) | 213 (359) |
Steel fiber, kg/m3 (lb/yd3) | 25 (42) |
Air-entraining admixture, mL/m3 (fl oz/yd3) | 400 (10.3) |
Water-reducing admixture, mL/m3 (fl oz/yd3) | 400 (10.3) |
Table 2:
Bekaert fiber properties in Mixtures A, B, and C
Bekaert fiber properties in Mixtures A, B, and C
Fiber properties | Mixture A | Mixture B | Mixture C |
Type | Dramix 3D-45/35 BL | Dramix 4D-65/35 BG | Dramix 5D-65/60 BG |
Length, mm (in.) | 35 (1.4) | 35 (1.4) | 60 (2.4) |
Aspect ratio | 45 | 65 | 65 |
Tensile strength, MPa (psi) | 1225 (177,700) | 1850 (268,300) | 2300 (333,600) |
Anchoring system | 3-face hook | 4-face hook | 5-face hook |
Table 3:
Summarized results from properties at fresh state and hardened state (28 days)
Summarized results from properties at fresh state and hardened state (28 days)
Properties | Mixture A | Mixture B | Mixture C |
Slump per ASTM C143/C143M, mm (in.) | 120 (4.75) | 100 (4.00) | 55 (2.25) |
Air content per ASTM C231/C231M before pumping and spraying, % | 6.8 | 9.4 | 7.4 |
Average compressive strength per ASTM C1604/C1604M, MPa (psi) | 49.0 (7110) | 45.4 (6580) | 51.7 (7500) |
Average peak load per ASTM C1550, N | 29,600 | 27,100 | 30,420 |
Average energy absorption per ASTM C1550, J | 5 mm (0.2 in.) deflection | 90 | 101 |
10 mm (0.4 in.) deflection | 115 | 183 | 245 |
20 mm (0.8 in.) deflection | 244 | 300 | 409 |
40 mm (1.6 in.) deflection | 349 | 456 | 597 |
Average peak load per EN 14488-511, kN | 61 | 67 | 91 |
Average energy absorption per EN 14488-511 at 25 mm (1 in.) deflection, J | 1010 | 1470 | 2120 |

Material | Quantity |
Cement, kg/m3 (lb/yd3) | 377 (635) |
Silica fume, kg/m3 (lb/yd3) | 29 (49) |
Fly ash, kg/m3 (lb/yd3) | 72 (121) |
Fine aggregate, kg/m3 (lb/yd3) | 1060 (1787) |
Coarse aggregate, kg/m3 (lb/yd3) | 568 (957) |
Water, kg/m3 (lb/yd3) | 213 (359) |
Steel fiber, kg/m3 (lb/yd3) | 25 (42) |
Air-entraining admixture, mL/m3 (fl oz/yd3) | 400 (10.3) |
Water-reducing admixture, mL/m3 (fl oz/yd3) | 400 (10.3) |
Fig. 2: Relative energy absorption values from ASTM C1550 and EN 14488-5 testing at maximum deflection for FRS with three different fibers at the same dosage
method should represent the actual loading conditions in which FRS will be used to truly evaluate its performance. It also means that, once a test method has been chosen for a project, it should be the only test method used throughout the entire project, from the initial design of the mixture to the quality control on-site—unless a clear correlation has been identified for a specific mixture.
Obviously, difficulties arise when the time comes to select an appropriate test method to work with. Before doing so, the engineer must not only reflect on the objective(s) of the test (including design, quality assurance, quality control, and research and development) but also identify a test method that will allow the engineer to truly discriminate between successful and meaningful results.
method should represent the actual loading conditions in which FRS will be used to truly evaluate its performance. It also means that, once a test method has been chosen for a project, it should be the only test method used throughout the entire project, from the initial design of the mixture to the quality control on-site—unless a clear correlation has been identified for a specific mixture.
Obviously, difficulties arise when the time comes to select an appropriate test method to work with. Before doing so, the engineer must not only reflect on the objective(s) of the test (including design, quality assurance, quality control, and research and development) but also identify a test method that will allow the engineer to truly discriminate between successful and meaningful results.
Conclusions
There is no doubt that FRS is applied using a unique placement process that yields a complex material. The rheology of the fresh shotcrete, the pumping aspects, and the consolidation process are all examples of what influences the in-place material. Although a good understanding is required to design and specify shotcrete, the knowledge is fortunately there for us to use. Besides, the complexity surrounding FRS is what makes it so versatile and useful. Indeed, when using the proper tools and materials, the possibilities are endless. Therefore, it is essential to use the information that is available and give FRS the consideration that it deserves.
Many challenges we must overcome remain, but this is how we will be able to use this effective tool at its full potential.
There is no doubt that FRS is applied using a unique placement process that yields a complex material. The rheology of the fresh shotcrete, the pumping aspects, and the consolidation process are all examples of what influences the in-place material. Although a good understanding is required to design and specify shotcrete, the knowledge is fortunately there for us to use. Besides, the complexity surrounding FRS is what makes it so versatile and useful. Indeed, when using the proper tools and materials, the possibilities are endless. Therefore, it is essential to use the information that is available and give FRS the consideration that it deserves.
Many challenges we must overcome remain, but this is how we will be able to use this effective tool at its full potential.
Acknowledgments
The authors would like to acknowledge the financial support of Bekaert, Natural Sciences and Engineering Research Council of Canada
(NSERC), and Fonds de Recherche du Québec—Nature et Technologies (FRQNT).
The authors would like to acknowledge the financial support of Bekaert, Natural Sciences and Engineering Research Council of Canada
(NSERC), and Fonds de Recherche du Québec—Nature et Technologies (FRQNT).
References
- Bentur, A., and Mindess, S., Fibre Reinforced Cementitious Composites, second edition, CRC Press, Boca Raton, FL, 2006, 624 pp.
- Armelin, H.S., and Helene, P., “Physical and Mechanical Properties of Steel-Fiber Reinforced Dry-Mix Shotcrete,” ACI Materials Journal, V. 92, No. 3, May-June 1995, pp. 258-267.
- “Guideline on the Applicability of Fibre-Reinforced Shotcrete for Ground Support in Mines,” MIGS III WP 24 Report, Nordic Rock Tech Centre, 2019, 53 pp.
- Soroushian, P., and Lee, C.-D., “Distribution and Orientation of Fibers in Steel Fiber Reinforced Concrete,” ACI Materials Journal, V. 87, No. 5, Sept.-Oct. 1990, pp. 433-439.
- Vandewalle, M., “Use of Steel Fibre Reinforced Shotcrete for the Support of Mine Openings.” Journal of the South African Institute of Mining and Metallurgy, V. 98, No. 3, May/June 1998, pp. 113-120.
- Burns, D., “Characterization of Wet-Mix Shotcrete for Small Line Pumping,” MS thesis, Department of Civil Engineering, Université Laval, Québec City, QC, Canada, 2008, 119 pp.
- Jolin, M.; Melo, F.; Bissonnette, B.; Power, P.; and Demmard, E., “Evaluation of Wet-Mix Shotcrete Containing Set-Accelerator and Service Life Prediction,” Proceedings: Shotcrete for Underground Support XII, M. Lu, O. Sigl, and G. Li, eds., Singapore, Oct. 2015, 13 pp.
- ACI Committee 506, “Guide to Fiber-Reinforced Shotcrete (ACI 506.1R-08),” American Concrete Institute, Farmington Hills, MI, 2008, 14 pp.
- Jolin, M.; Lemay, J.-D.; Ginouse, N.; Bissonnette, B.; and Blouin- Dallaire, É., “The Effect of Spraying on Fiber Content and Shotcrete Properties,” Proceedings: Shotcrete for Underground Support XII, M. Lu, O. Sigl, and G. Li, eds., Singapore, Oct. 2015, 15 pp.
- UNE 83515:2010, “Hormigones con Fibras. Determinación de la Resistencia a Fisuración, Tenacidad y Resistencia Residual a Tracción. Método Barcelona” (Fibre Reinforced Concrete. Determination of Cracking Strength, Ductility and Residual Tensile Strength. Barcelona Test), Asociación Española de Normalización y Certificación (AENOR), Madrid, Spain, 2010, 10 pp.
- EN 14488-5, “Testing Sprayed Concrete—Part 5: Determination of Energy Absorption Capacity of Fibre Reinforced Slab Specimens,” European Committee for Standardization, Brussels, Belgium, 2006, 10 pp.
- EN 14651, “Test Method for Metallic Fibre Concrete. Measuring the Flexural Tensile Strength (Limit of Proportionality [LOP], Residual)” European Committee for Standardization, Brussels, Belgium, 2007, 19 pp.
- “EFNARC Three Point Bending Test on Square Panel with Notch,” EFNARC, June 2011, 12 pp.
- “Publication No. 7: Sprayed Concrete for Rock Support,” Norwegian Concrete Association, Oslo, Norway, 2011, 103 pp.
- Grimstad, E., and Barton, N., “Updating the Q-System for NMT,” Sprayed Concrete: Modern Use of Wet Mix Sprayed Concrete for Underground Support, R. Kompen, O. Opsahl, and K. Berg, eds., International Symposium on Sprayed Concrete, Fagernes, Norway, Oct. 1993, 464 pp.
- ACI Committee 506, “Guide to Shotcrete (ACI 506R-16),” American Concrete Institute, Farmington Hills, MI, 2016, 52 pp.
- ACI Committee 544, “Report on the Measurement of Fresh State Properties and Fiber Dispersion of Fiber-Reinforced Concrete (ACI 544.2R-17),” American Concrete Institute, Farmington Hills, MI, 2017, 20 pp.
- ACI Committee 544, “Guide for Specifying, Proportioning, and Production of Fiber-Reinforced Concrete (ACI 544.3R-08),” American Concrete Institute, Farmington Hills, MI, 2008, 12 pp.
- ACI Committee 544, “Guide to Design with Fiber-Reinforced Concrete (ACI 544.4R-18),” American Concrete Institute, Farmington Hills, MI, 2018, 39 pp.
- ACI Committee 544, “Report on the Physical Properties and Durability of Fiber-Reinforced Concrete (ACI 544.5R-10),” American Concrete Institute, Farmington Hills, MI, 2010, 31 pp.
- ACI Committee 544, “Report on Design and Construction of Steel Fiber-Reinforced Concrete Elevated Slabs (ACI 544.6R-15),” American Concrete Institute, Farmington Hills, MI, 2015, 38 pp.
- ACI Committee 544, “Report on Indirect Method to Obtain Stress- Strain Response of Fiber-Reinforced Concrete (FRC) (ACI 544.8R-16),” American Concrete Institute, Farmington Hills, MI, 2016, 22 pp.
- ACI Committee 544, “Report on Measuring Mechanical Properties of Hardened Fiber-Reinforced Concrete (ACI 544.9R-17),” American Concrete Institute, Farmington Hills, MI, 2017, 48 pp.
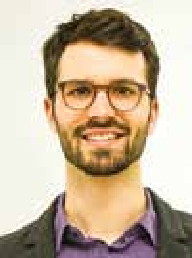
ACI member Antoine Gagnon is a PhD Student in the Department of Civil and Water Engineering at Université Laval, Québec City, QC, Canada. His research focuses on the development of tools for the design and testing of fiber-reinforced shotcrete for ground support. He has worked on shotcrete research projects with different companies in the industry. He is Chair of ACI Subcommittee 506-B,
Shotcreting-Fiber-Reinforced, and a member of ACI Committees
506, Shotcreting, and 544, Fiber Reinforced Concrete. He received his bachelor’s degree and his master’s degree in civil engineering from Université Laval.
Shotcreting-Fiber-Reinforced, and a member of ACI Committees
506, Shotcreting, and 544, Fiber Reinforced Concrete. He received his bachelor’s degree and his master’s degree in civil engineering from Université Laval.

Marc Jolin, FACI, is a Professor in the Department of Civil and Water Engineering at Université Laval. He is Chair of ACI Subcommittee 506-L, Liaison Subcommittee; past Chair of
ACI Committees 506, Shotcreting, and C660, Shotcrete Nozzleman Certification; Secretary of ACI Committee C661, Shotcrete Inspector Certification; and an ACI Examiner for Shotcrete Nozzleman Certification (wet- and dry-mix processes). Jolin is also a member of the American Shotcrete Association. He received his PhD from the University of British Columbia, Vancouver, BC, Canada
ACI Committees 506, Shotcreting, and C660, Shotcrete Nozzleman Certification; Secretary of ACI Committee C661, Shotcrete Inspector Certification; and an ACI Examiner for Shotcrete Nozzleman Certification (wet- and dry-mix processes). Jolin is also a member of the American Shotcrete Association. He received his PhD from the University of British Columbia, Vancouver, BC, Canada
06 Sep 2021